Overview of Role of HLA Matching
Of all the genetic and non-genetic factors that can influence stem cell transplant outcome, the most important remains HLA matching.
HLA matching is required for all forms of stem cell transplantation other than autologous transplantation. Matching is required at all the classical HLA class I and II loci, HLA-A, B and C, HLA-DRB1, DQB1 and HLA-DPB1 at high resolution allele level. Graft versus Host Disease (GvHD) is a major post-transplant complication in stem cell transplantation and can be initiated by as little as a single amino acid mismatch in the peptide binding groove of an HLA antigen. The degree of matching required will be influenced by the nature of the disease and the source of the stem cells. Studies by Petersdorf et. al., and others have shown that the risk of GvHD increases with increasing numbers of mismatches in the Graft versus Host direction (i.e. HLA alleles present in the patient but absent in the donor). Similar studies have also shown that the risk of graft failure increases with increasing numbers of mismatches in the Host versus Graft direction (i.e. HLA alleles present in the donor but absent in the patient).
In autologous stem cell transplantation, the donor is the patient, making the HLA alleles the same. Any HLA typing undertaken is therefore not for matching for the transplant but rather for information should an allogeneic donor suddenly be needed or for supportive therapy such as the use of HLA matched platelets.
In the allogeneic setting, HLA matching is required at the high-resolution allele level, the ‘best’ matched donor being a syngeneic or genetically identical donor who will be allele matched at all the classical HLA loci. However, whilst the absence of HLA mismatches in the syngeneic setting reduce the risk of GvHD, it also reduces the desirable Graft versus Leukaemia (GvL) effect and is therefore associated with an increased incidence of relapse. In the absence of a syngeneic donor, a HLA matched sibling represents the most likely source of a HLA matched donor. A sibling donor has a ¼ chance of being HLA matched. Historically, some Transplant Centres limited their HLA typing to the low to medium resolution HLA typing i.e. first field DNA typing, at HLA-A, B and DR as sufficient to confirm a match between and patient and a sibling donor because of the strong linkage disequilibrium between HLA-B and C as well as between HLA-DR and DQ. This is the minimum required by European Federation for Immunogenetics (EFI) standards in related stem cell transplantation. However limiting typing to these loci may mean that the patient and donor may not be matched at HLA-C and HLA-DQ if one or more parents are homozygous for HLA-A, B, DR as the patient and donor could inherit different haplotypes which could be mismatches at the allele level when high resolution or second field typing is undertaken. There could also be crossover events between HLA-A and HLA-B and between HLA-DR/DQ and HLA-DP which would remain undetected if typing is not extended to all the classical HLA genes. For this reason, when matching siblings, it is also useful to determine the HLA types of the parents if possible. In addition, where parents share a haplotype, a parent could be matched to the patient and represent a potential source of stem cells. Historically, HLA-DPB1 matching was not always taken into account though has now largely changed.
For unrelated allogeneic transplantation with adult Peripheral Blood Stem Cells (PBSC) or Bone Marrow (BM), HLA matching was historically undertaken for HLA-A, B, C, DR and DQ at the high resolution second field level. A large study by the NMDP indicated that 8/8 matching, excluding HLA-DQB1, has the same outcome as 10/10 matching and is the minimum required for stem cell transplantation. Matching for HLA-DPB1 is now taken into account by many transplant centres though HLA-DPB1 mismatching is associated with increased GvL and therefore a lower risk of relapse making a degree of HLA-DPB1 mismatching desirable in some malignant diseases.
Transplantation with Cord Blood (CB) derived stem cells leads to a reduced incidence and severity of GvHD due to the relative immaturity of the immune system at birth. This can allow less stringent HLA matching for CB transplantation with one or two HLA mismatches tolerated. CB matching is carried out at high resolution at HLA-A, B, C and DR for a single cord. For double cord, matching is currently at the broad antigen level for class I and at the allele level for HLA-DR. HLA mismatches between the cord blood units is no longer considered to be relevant. Cell dose may be the more important factor.
The use of cord blood helps in the identification of donors for patients with rarer HLA types or uncommon haplotypes. Registry data does indicate that cord blood banks have a higher frequency of uncommon phenotypes than adult bone marrow registries.
The relative importance of matching at the individual classical HLA loci in stem cell transplant outcome is controversial with many apparently contradicting studies. This may be due to the wide range of disease groups, conditioning regimes and HLA typing techniques used over the years. Some studies have shown that in high-risk patients, transplantation with a donor with a single HLA allele mismatch leads to a better outcome than a prolonged search for a fully matched donor. An additive effect appears to exist such that the total number of mismatches may be more important than which specific loci are mismatched, though many transplant teams would prefer to avoid mismatches at class II (especially HLA-DRB1) over mismatches at class I.
Both HLA and non-HLA genes play a role in stem cell transplant outcome and therefore influence donor selection. HLA genes are the main genetic barrier to transplantation and therefore have the most influence on donor selection but non-HLA genes play a significant and increasingly understood role.
Relevance of HLA Matching at the Allele Level
High resolution typing refers to a level of typing which is able to assign HLA alleles on the basis of differences in exons 2 and 3 for class I and exon 2 for class II. Alleles with identical sequences over these exons are all reported as part of a high-resolution HLA typing result though further testing over additional exons is often performed to further reduce ambiguities.
Allele level typing on the other hand refers to the unambiguous identification of the allele present.
Until very recently, most H&I laboratories performed high-resolution HLA typing by Sanger-based Sequencing (SBT). SBT has some limitations as it is not always able to resolve ambiguities. It often requires additional testing with group specific sequencing primers (GSSP) to help resolve these ambiguities but some do still remain. Recently, NGS techniques have been introduced. These tend to have much fewer ambiguities and superior phasing depending on the technique used, whole gene sequencing on the one hand versus shotgun sequencing which can have a small number of ambiguities.
The clinical advantages of high-resolution HLA matching over and above low to intermediate HLA matching in stem cell transplantation have been clearly demonstrated in reduced GvHD and improved overall survival. There is insufficient data at present to clearly define a clinical advantage of allele level HLA matching in stem cell transplantation over and above high-resolution HLA matching. The Anthony Nolan have some recently published data which shows superior one year mortality and morbidity and reduced GvHD for allele level matching compared to high resolution matching, especially when HLA-DPB1 was included in a 12/12 match. Potential reasons for this include:
- Allele level matching resolves remaining ambiguities relative to HR
- Allele level matching reduces variations in introns and untranslated regions which may be significant
- Allele level matching may be a surrogate for reduced SNP mismatches in other regions
Relevance of ‘G’ and ‘P’ code in HSCT
G code alleles are those HLA alleles that have identical nucleotide sequences across the exons encoding the peptide binding domains (exon 2 and 3 for HLA class I and exon 2 only for HLA class II alleles) while P code alleles include all those with, for HLA class I, identical protein sequences encoded by exons 2 and 3 and for HLA Class II, identical protein sequences as encoded by exon 2.
Examples of alleles that share the same G code include DQB1*03:01:01:01 with DQB1*03:19:01:01 and C*05:01:01:01 with C*05:37. Examples of alleles that share the same P code include DRB1*14:01:01 with DRB1*14:54:01:01.
For unrelated donor transplants where a patient appears to only have a 11/12 match with the only mismatch being a G or P coded allele, one strategy could be to first get the probability of the patient being able to get a full 12/12 allele match using the WMDA, NMDP or allele frequency tools. If the patient has a low probability of getting a better match, a literature seach would be conducted to see if there are any published data concerning permissible mismatches using the particular G or P code. If there are no specific references for the mismatch in question, there are general publications outlining reduced risk of transplants where the mismatches are outside of exons 2 and 3 for class I and exon 2 for class II. In all transplants with G or P code alleles other additional risk factors such CMV mismatching should be avoided.
Role of HLA-DPB1 Matching
HSCT where HLA allele are matched 10/10 at HLA-A, -B, -C, -DRB1 and -DQB1 are mismatched at HLA-DPB1 in approximately 80% of cases and these mismatches have been shown to correlate significantly with non-relapse mortality. Finding donors matched 12/12 to include HLA-DPB1 can be difficult due to a recombination hotspot between HLA-DQ and -DP. However, the classification of HLA-DPB1 alleles into permissive and non-permissive mismatches based on T-cell-epitope groups increases the odds of funding an unrelated donor for a patient. Classification of HLA-DPB1 into permissive and therefore tolerated and non-permissive mismatches has been shown to be significant in unrelated-donor HSCT.
Zino et al demonstrated in a paper published in Blood in 2004, through a series of EBV transformed B cell line cytotoxic assays, that subsets of HLA-DPB1 alleles shared T cell epitopes that determine their specific allo-response.
They divided HLA-DPB1 alleles into three groups, group 1 – Immunogenic, group 2 – intermediately immunogenic and group 3 poorly immunogenic. They hypothesised that expression of a group 1 or 2 allele would lead to clonal deletion of T cells specific for the epitope on that allele. Donors who shared those group 1 or 2 alleles were therefore likely to be permissive. Expression of a group 3 allele was predicted not to induce negative selection of alloreactive T cells specific for the shared epitope.
Broadly speaking, where patients and donors shared alleles from the same groups, the mismatch is permissive. Where patient alleles are in different groups, the mismatch is non-permissive.
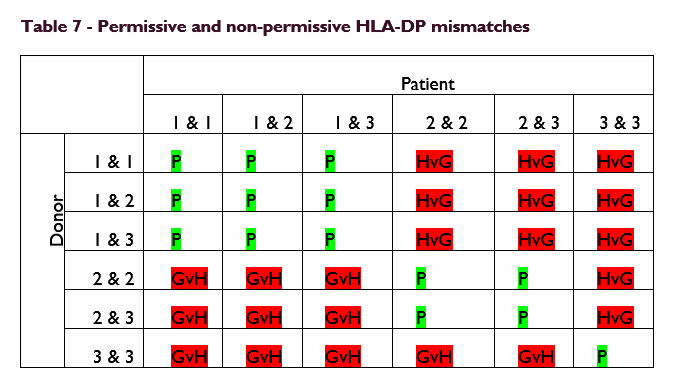
These data demonstrate that T cell epitope matching defines permissive and non-permissive HLA-DPB1 mismatching in HSCT. Selection of unrelated donors with permissive mismatches increases the donor options for stem cell patients.
Recently Petersdorf et al have proposed an additional model of HLA-DPB1 permissiveness based on the level of HLA-DPB1 expression due to a SNP in the 3’ Untranslated Region (UTR). In this model, a donor with a HLA-DPB1 type that is highly expressed has a higher aGvHD incidence and donors with low expression have lower incidence of aGvHD.
A third model is based on HLA-DPB1 types being classified into two evolutionarily defined groups.
Non-permissive HLA-DPB1 mismatches are associated with significantly higher risks of treatment-related mortality compared to permissive mismatches. However non-permissive mismatches in the graft versus host (GvH) direction also significantly decreased the risk of relapse compared to permissive mismatches because of the GvL effect.
Null HLA Alleles
HLA null alleles are alleles for which no HLA products are expressed at the cell surface. Over 1,200 null alleles have been described across HLA class I and II (Mar 2021). Examples include A*01:04:01:01N and A*01:11N. The nomenclature for null alleles includes the ‘N’ suffix symbolising null. Other alleles which are alternatively expressed have the suffixes ‘L’ for low expression of HLA product, ‘S’ for products which are secreted rather than being expressed on the cell surface, ‘C’ for product found only in the cytoplasm, ‘A’ for product with aberrant expression where there is some doubt about the cell surface expression and ‘Q’ for products with questionable expression, where the mutation in the allele has previously been shown to affect normal expression.
Mutations in allele sequences which lead to null alleles include insertions, deletions and point mutation many of which lead to the introduction of stop codons, though some lead to the development of incorrectly spliced products. Many of these mutations are located outside of exons 2 and 3 for class I and outside of exon 2 for class II. DNA based HLA typing techniques that do not inspect the gemone outside of these regions therefore run a risk of misidentifying null alleles. Misidentifying a null allele for its fully expressed counterpart poses a significant risk in stem cell transplantation.
If a stem cell donor null allele is misidentified as a fully expressed product and therefore transplanted into a patient bearing the expressed antigen, the patient’s antigen will be allogeneic for the donor’s T cells and can lead to severe acute GvHD. In the reverse scenario, if a patient null allele is misidentified as a fully expressed product and therefore transplanted with a donor bearing the expressed antigen, the donors’ antigen will be allogeneic for the patients’ T cells and can lead to destruction of the donor stem cells and graft failure.
Donor selection for stem cell transplantation does not typically take DRB3, 4 and 5 into account. Patients and donors matched at HLA-DRB1 and DQB1 are also typically matched at DRB3, 4 and 5. Where a patient is DRB4*02:01N (al DR53 allele) for instance, provided patient and donor are matched at HLA-DRB1 and DQB1, the donor is also very likely to also be DRB4*02:01N. However, this needs to be checked, especially if DQB1 matching is not take into account, as transplanting a DR53 Pos. donor into this patient carries a risk of graft rejection. DRB3, 4 and 5 may also need to be undertaken fi the patient has antibodies directed against DRB3, 4 and 5.
Various studies have shown that low expressed alleles are expressed in sufficient numbers to result in T cell tolerance which means that in a stem cell transplant, misidentification of low expressed alleles in either the donor or the patient is likely to be tolerated.
Compared to the impact in stem cell transplantation, misidentification of null alleles in solid organ transplantation potentially has a reduced clinical impact. If a patient null allele is misidentified as a fully expressed product and therefore transplanted with a donor bearing the expressed antigen, this results in a mismatch which does not have a clinical consequence provided the patient does not have HLA antibodies directed against the mismatch. The patient does however have a risk of developing donor specific antibodies for that mismatch. A donor null allele misidentified as a fully expressed product and therefore transplanted into a patient bearing the expressed antigen results in no humoral rejection and is well tolerated.
In the case of low expressed alleles, misidentifying a low expressed allele for its fully expressed variant presents very little risk to in solid organ transplantation. Even overlooking a low expressed allele such as HLA-A*24:01:01:02L in a donor carries only a small risk of humoral rejection by preformed anti-A24 antibodies due to the low expression.
A Putative New Allele
Discovery of a putative new allele in a stem cell patient means that the patient is very unlikely to be able to obtain a 12/12 unrelated donor. The first step upon discovering a putative new allele is to repeat and confirm the sequence using a buccal cavity sample to rule our disease driven mutations.
For a patient with a putative new allele that has a matched family donor, transplant can proceed with the related donor following confirmation that the sequences of the patient and potential donor are the same.
Where a matched related donor is not available, the decision on how to proceed to transplant will depend on the disease and the preferences/experience of the transplant centre. For diseases where mismatched transplants are indicated, some centres may prefer a related haplo donor over a 11/12 unrelated donor. If using a 11/12 unrelated donor, potentially permissible mismatches i.e. mismatches where the difference is outside exon 2 and 3 for class I or exon I for class II are preferred. Antibody screening of the patient is required to make sure the patient does not have HLA antibodies directed against the donor mismatches.
In all cases the sequence of the putative new allele must be submitted to the nomenclature committee for confirmation and assignment of an allele name.